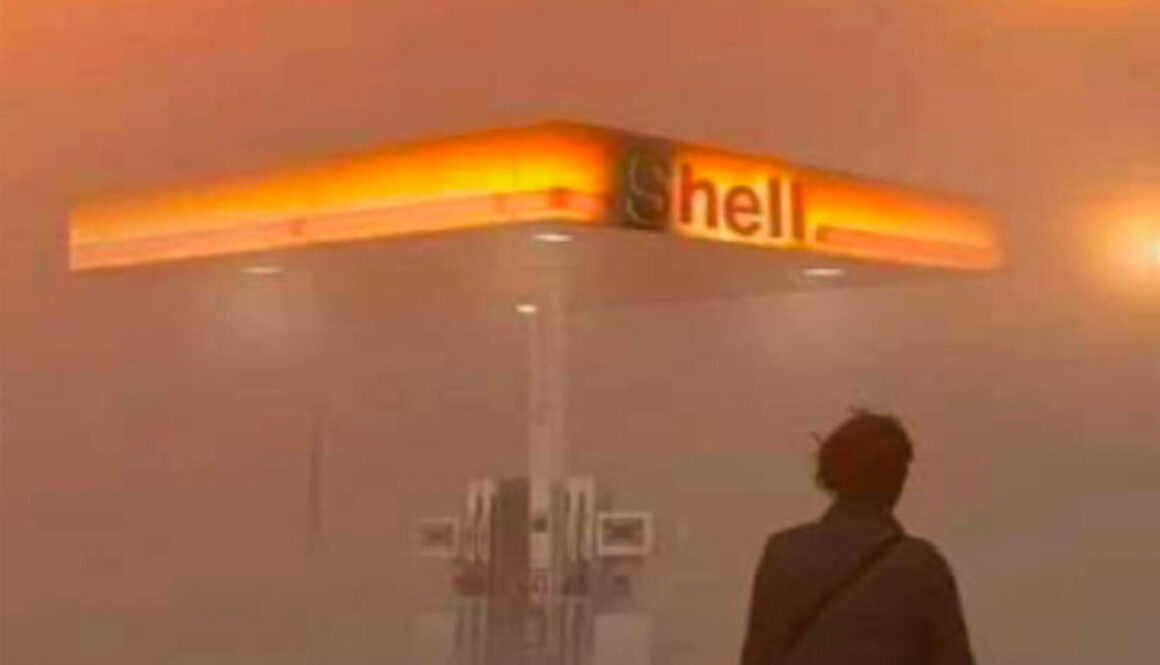
heat
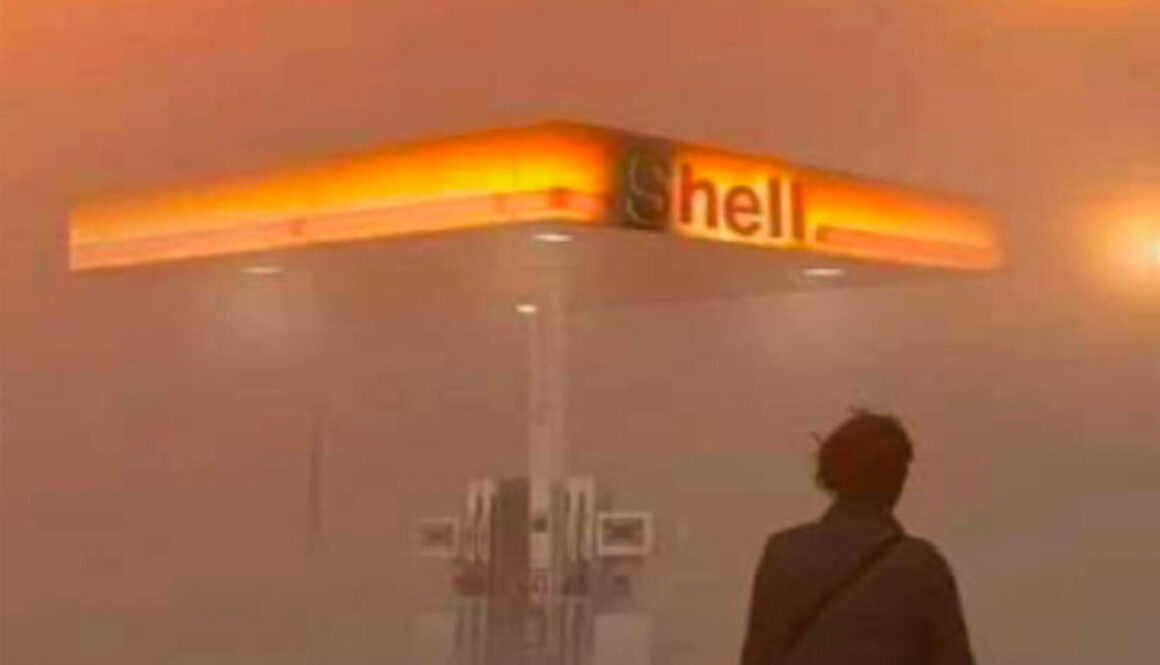
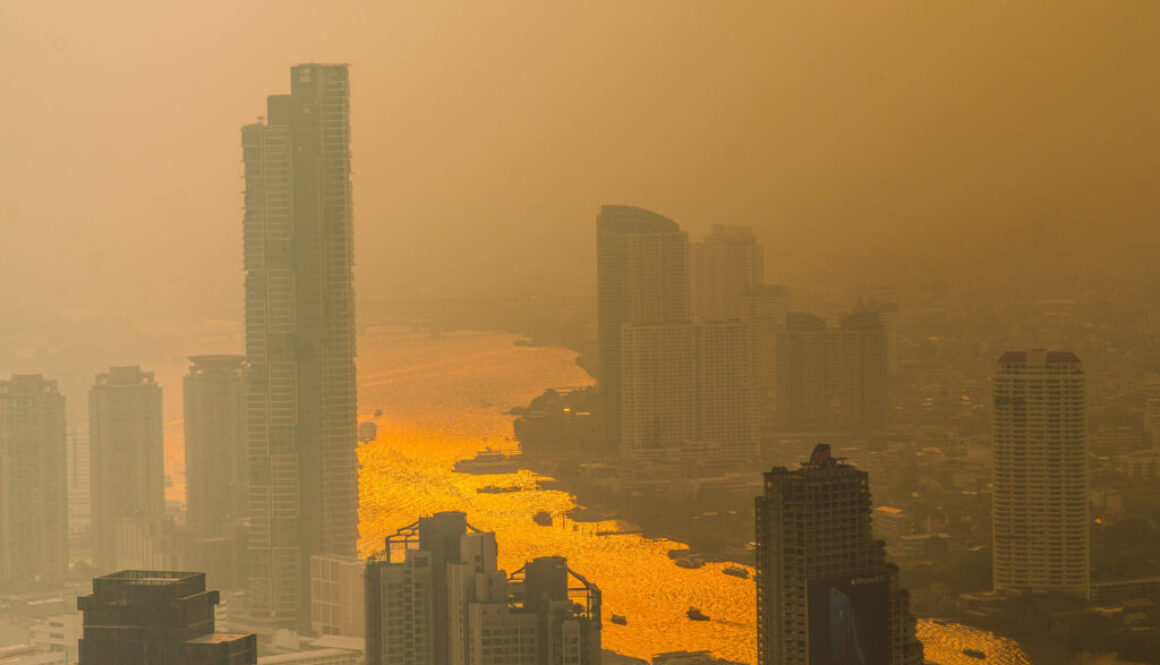
This article is republished from The Conversation under a Creative Commons license. Read the original article.
___________________________________________________________________________________
The world is striving to reach net-zero emissions as we try to ward off dangerous global warming. But will getting to net-zero actually avert climate instability, as many assume?
Our new study examined that question. Alarmingly, we found reaching net-zero in the next few decades will not bring an immediate end to the global heating problem. Earth’s climate will change for many centuries to come.
And this continuing climate change will not be evenly spread. Australia would keep warming more than almost any other land area. For example if net-zero emissions are reached by 2060, the Australian city of Melbourne is still predicted to warm by 1°C after that point.
But that’s not to say the world shouldn’t push to reach net-zero emissions as quickly as possible. The sooner we get there, the less damaging change the planet will experience in the long run.
Reaching net-zero is vital
Global greenhouse gas emissions hit record highs in 2023. At the same time, Earth experienced its hottest year.
Analysis suggests emissions may peak in the next couple of years then start to fall. But as long as emissions remain substantial, the planet will keep warming.
Most of the world’s nations, including Australia, have signed up to the Paris climate agreement. The deal aims to keep global warming well below 2°C, and requires major emitters to reach net-zero as soon as possible. Australia, along with many other nations, is aiming to reach the goal by 2050.
Getting to net-zero essentially means nations must reduce human-caused greenhouse gas emissions as much as possible, and compensate for remaining emissions by removing greenhouse gases from the atmosphere elsewhere. Methods for doing this include planting additional vegetation to draw down and store carbon, or using technology to suck carbon out of the air.
Getting to net-zero is widely considered the point at which global warming will stop. But is that assumption correct? And does it mean warming would stop everywhere across the planet? Our research sought to find out.
Centuries of change
Computer models simulating Earth’s climate under different scenarios are an important tool for climate scientists. Our research used a model known as the Australian Community Climate and Earth System Simulator.
Such models are like lab experiments for climate scientists to test ideas. Models are fed with information about greenhouse gas emissions. They then use equations to predict how those emissions would affect the movement of air and the ocean, and the transfer of carbon and heat, across Earth over time.
We wanted to see what would happen once the world hit net-zero carbon dioxide at various points in time, and maintained it for 1,000 years.
We ran seven simulations from different start points in the 21st century, at five-year increments from 2030 to 2060. These staggered simulations allowed us to measure the effect of various delays in reaching net-zero.
We found Earth’s climate would continue to evolve under all simulations, even if net-zero emissions was maintained for 1,000 years. But importantly, the later net-zero is reached, the larger the climate changes Earth would experience.
Warming oceans and melting ice
Earth’s average temperature across land and sea is the main indicator of climate change. So we looked at that first.
We found this temperature would continue to rise slowly under net-zero emissions – albeit at a much slower rate than we see today. Most warming would take place on the ocean surface; average temperature on land would only change a little.
We also looked at temperatures below the ocean surface. There, the ocean would warm strongly even under net-zero emissions – and this continues for many centuries. This is because seawater absorbs a lot of energy before warming up, which means some ocean warming is inevitable even after emissions fall.
Over the last few decades of high greenhouse gas emissions, sea ice extent fell in the Arctic – and more recently, around Antarctica. Under net-zero emissions, we anticipate Arctic sea ice extent would stabilise but not recover.
In contrast, Antarctic sea ice extent is projected to fall under net-zero emissions for many centuries. This is associated with continued slow warming of the Southern Ocean around Antarctica.
Importantly, we found long-term impacts on the climate worsen the later we reach net-zero emissions. Even just a five-year delay would affect on the projected climate 1,000 years later.
Delaying net-zero by five years results in a higher global average surface temperature, a much warmer ocean and reduced sea ice extent for many centuries.
Australia’s evolving climate
The effect on the climate of reaching net-zero emissions differs across the world.
For example, Australia is close to the Southern Ocean, which is projected to continue warming for many centuries even under net-zero emissions. This warming to Australia’s south means even under a net-zero emissions pathway, we expect the continent to continue to warm more than almost all other land areas on Earth.
For example, the models predict Melbourne would experience 1°C of warming over centuries if net-zero was reached in 2060.
Net-zero would also lead to changes in rainfall in Australia. Winter rainfall across the continent would increase – a trend in contrast to drying currently underway in parts of Australia, particularly in the southwest and southeast.
Knowns and unknowns
There is much more to discover about how the climate might behave under net-zero.
But our analysis provides some clues about what climate changes to expect if humanity struggles to achieve large-scale “net-negative” emissions – that is, removing carbon from the atmosphere at a greater rate than it is emitted.
Experiments with more models will help improve scientists’ understanding of climate change beyond net-zero emissions. These simulations may include scenarios in which carbon removal methods are so successful, Earth actually cools and some climate changes are reversed.
Despite the unknowns, one thing is very clear: there is a pressing need to push for net-zero emissions as fast as possible.
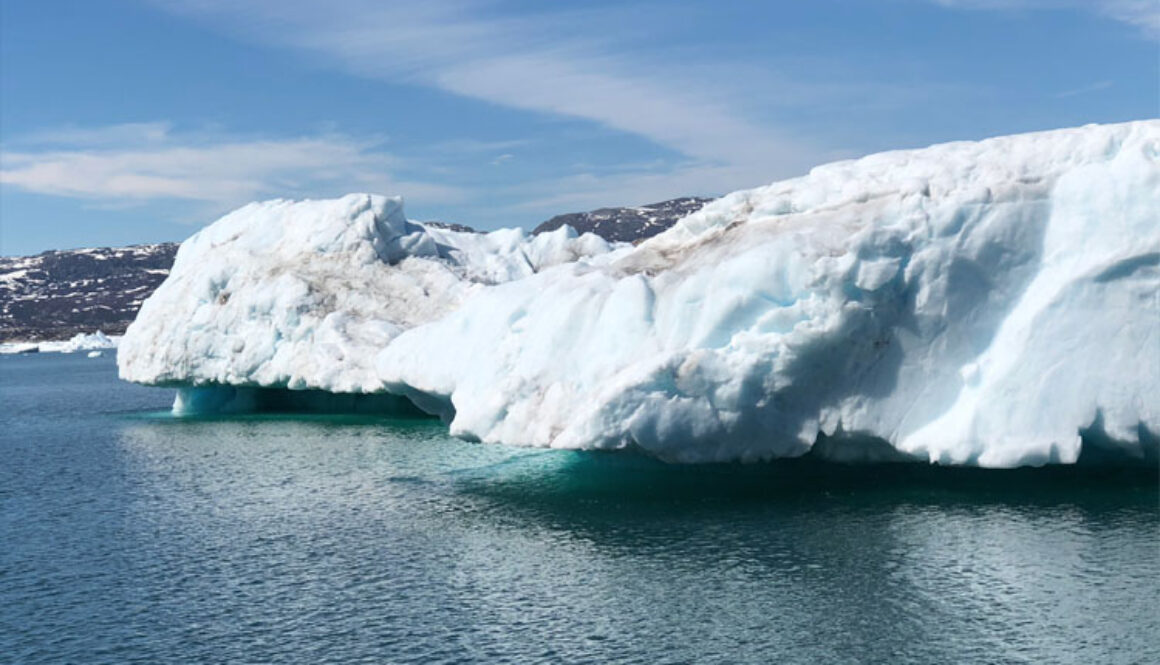
Recent assessments suggest the ocean current known as Atlantic Meridional Overturning Circulation (AMOC) is slowing down, with collapse a real possibility this century.
The AMOC is a globally important current in the Atlantic Ocean, where surface water moves northward as part of the Gulf Stream and transports warm water towards the Arctic. There it cools and sinks to return southward as a deep ocean current.

Collapse of the AMOC would have a devastating effect on climate in Europe. Temperatures in the UK and Scandinavia could drop by 5–15°C in a matter of decades.
However, because Earth’s climate system is interconnected, these impacts could have a global reach. Our new research shows past changes in AMOC have had significant impact on temperatures in New Zealand and across the southern hemisphere. These results imply that future collapse of AMOC may accelerate ongoing warming trends.
Lessons from the past
Between 20,000 and 10,000 years ago, Earth transitioned from peak ice-age conditions to a climate more like today’s. This interval featured rising global temperatures, melting ice sheets and climbing sea levels – all phenomena associated with present-day climate change.
Evidence from ice cores in Greenland and marine sediments in the North Atlantic suggests this natural warming event featured several abrupt changes associated with AMOC variability.
Using this interval as a natural experiment, we have undertaken research to learn more about how AMOC variability can affect climate in New Zealand.

To reconstruct how air temperature changed in New Zealand, we examined the past extent of mountain glaciers using evidence preserved in the landscape. Glaciers grow and shrink primarily in response to changing air temperature, which affects the annual balance of snowfall and snow or ice melt. As glaciers change in size, they deposit moraines (rock debris) in the landscape, which can persist for tens of thousands of years.

We combined these land-based observations with reconstructions of sea-surface temperature in the Tasman Sea, which we derived from microfossils (smaller than one millimetre in size) known as foraminifera. These microfossils come in a wide range of species and each has a preferred water temperature.
We quantified changes in foraminifera species in a core of marine sediment to trace how local temperature in the Tasman Sea has varied through time.
Global climate connections
Our results show that changes in air and sea-surface temperature followed a similar pattern in the New Zealand region as Earth warmed following the last ice age.
Warming began in both air and sea at about 18,000 years ago, followed by a cooling event at about 14,500 years ago – the Antarctic Cold Reversal. The timing of these changes matches past changes in the AMOC, as recorded in geological climate records from the North Atlantic region.
We examined computer simulations to test the physical connection between changes in the AMOC and New Zealand’s climate. These simulations used a physics-based climate model that captures atmospheric and ocean circulation and their interaction.

The model simulations support our geological evidence, showing air and sea surface temperatures in New Zealand respond sensitively to changes in AMOC intensity. When the AMOC weakens and Europe cools, New Zealand and the southern mid-latitudes undergo warming, and vice versa.
The models also indicate changes in the AMOC are transported rapidly, within decades, to New Zealand via shifting global wind systems. Changes in the AMOC disrupt the temperature gradient between the hemispheres, which is a key control on the strength of westerly wind belts in the southern hemisphere, between the latitudes of 40°S and 60°S where New Zealand is.
The westerly winds are important for New Zealand’s climate. They control the path of atmospheric storms and regional ocean currents.
Stronger winds over New Zealand bring regional cooling, as more storms track over the country and warm ocean currents are diverted away from the Tasman Sea into the south Pacific. In contrast, when the AMOC weakens, New Zealand has clearer skies and the Tasman Sea receives more tropical water masses, causing regional warming.
Future implications
Scientists have identified several “tipping points” in Earth’s climate system that may be triggered by human-caused climate change. Once these thresholds are crossed, the consequences cannot be easily undone.
Climbing greenhouse gas concentrations have raised air temperatures in New Zealand, and globally, by about 1.1°C since the late 19th century. Projections suggest New Zealand may end this century 1°C to 3°C warmer than now. However, these estimates do not include the potential impacts of a future AMOC collapse.
Our insights from the recent geological past show this AMOC tipping point has global reach, and could accelerate future warming in New Zealand.
Shaun Eaves, Senior Lecturer in Physical Geography, Te Herenga Waka — Victoria University of Wellington; Andrew Mackintosh, Chief Investigator in Antarctic environmental science, Monash University; Helen Bostock, Associate Professor in Marine Geology, The University of Queensland, and Joel Pedro, Palaeoclimatologist
This article is republished from The Conversation under a Creative Commons license. Read the original article.
Authors: Andrew Dowdy, The University of Melbourne; Conrad Wasko, University of Sydney; Jennifer Catto, University of Exeter, and Seth Westra, University of Adelaide
In media articles about unprecedented flooding, you’ll often come across the statement that for every 1°C of warming, the atmosphere can hold about 7% more moisture.
This figure comes from research undertaken by the French engineer Sadi Carnot and published 200 years ago this year.
We now know there’s more to the story. Yes, a hotter atmosphere has the capacity to hold more moisture. But the condensation of water vapour to make rain droplets releases heat. This, in turn, can fuel stronger convection in thunderstorms, which can then dump substantially more rain.
This means that the intensity of extreme rainfall could increase by much more than 7% per degree of warming. What we’re seeing is that thunderstorms can likely dump about double or triple that rate – around 14–21% more rain for each degree of warming.
Thunderstorms are a major cause of extreme flooding around the world, contributing to Brazil’s disastrous floods, which have submerged hundreds of towns, and Dubai’s flooded airport and roads.
For Australia, we helped develop a comprehensive review of the latest climate science to guide preparedness for future floods. This showed the increase per degree of global warming was about 7–28% for hourly or shorter duration extreme rain, and 2–15% for daily or longer extreme rain. This is much higher than figures in the existing flood planning standards recommending a general increase of 5% per degree of warming.
Why are thunderstorms important for extreme rain?
For thunderstorms to form, you need ingredients such as moisture in the air and a large temperature difference between lower and higher air masses to create instability.
We typically associate thunderstorms with intense localised rain over a short period. What we’re seeing now, though, is a shift towards more intense thunderstorm downpours, particularly for short periods.
Extreme rain events are also more likely when thunderstorms form in combination with other weather systems, such as east coast lows, intense low pressure systems near eastern Australia. The record floods which hit Lismore in February 2022 and claimed the lives of many people came from extreme rain over many days, which came in part from severe thunderstorms in combination with an east coast low.
Climate change pumps up extreme flood risk factors
The latest report from the Intergovernmental Panel on Climate Change (IPCC) states that:
frequency and intensity of heavy precipitation events have increased since the 1950s over most land areas for which observational data are sufficient for trend analysis (high confidence), and human-induced climate change is likely the main driver
This increase is particularly clear in short-duration extreme rains, such as those caused by thunderstorms.
Why? In part, it’s because of the 7% figure – warmer air is able to hold more water vapour.
But that doesn’t explain everything. There’s something else going on. Condensation produces heat. So as water vapour turns into droplets, more heat becomes available, and hot air rises by convection. In thunderstorms, more heat fuels stronger convection, where warm, moisture-laden air is driven up high.
This explains why thunderstorms can now drive such extreme rainfall in our warming world. As water vapour condenses to make rain, it also makes heat, supercharging storms.
We are seeing these very rapid rates of rainfall increase in recent decades in Australia.
Daily rainfall associated with thunderstorms has increased much more than the 7% figure would suggest – about 2-3 times more.
Hourly rainfall extremes have also increased in intensity at similar rates.
What about very sudden, extreme rains? Here, the rate of increase could potentially be even larger. One recent study examined extreme rain for periods shorter than one hour near Sydney, suggesting about a 40% increase or more over the past 20 years.
Rapid trends in extreme rainfall intensity are also clear in other lines of evidence, such as fine-resolution modelling.
To model complex climate systems, we need the grunt of supercomputers. But even so, many of our models for climate projections don’t drill down to grid resolutions smaller than about 100 kilometres.
While this can work well for large-scale climate modelling, it’s not suitable for directly simulating thunderstorms. That’s because the convection processes needed to make thunderstorms form happen on much smaller scales than this.
There’s now a concerted effort underway to perform more model simulations at very fine scales, so we can improve the modelling of convection.
Recent results from these very fine scale models for Europe suggest convection will play a more important role in triggering extreme rainfall including in combined storms, such as thunderstorms mingling with low pressure systems and other combinations.
This matches Australian observations, with a trend towards increased rain from thunderstorms combining with other storm types such as cold fronts and cyclones (including low-pressure systems in southern Australia).
Does this change how we plan for floods?
The evidence for supercharged thunderstorm rainfall has grown in recent years.
Australia’s current flood guidance recommendations, which influence how infrastructure projects have been built, are based on extreme rain increasing by just 5% for each degree of warming.
Our research review has shown the real figure is substantially higher.
This means roads, bridges, tunnels built for the 5% figure may not be ready to deal with extreme rain we are already seeing from supercharged thunderstorms.
While Australia has become more conscious of links between climate change and bushfires, studies show we are less likely to link climate change and more intense storms and floods.
This will have to change. We still face some uncertainties in precisely linking climate change to a single extreme rain event. But the bigger picture is now very clear: a hotter world is likely one with higher risk of extreme floods, often driven by extreme rain from supercharged thunderstorms.
So what should we do? The first step is to take climate change influences on storms and flood risk as seriously as we now do for bushfires.
The next is to embed the best available evidence in how we plan for these future storms and floods.
We have already loaded the dice for more extreme floods, due to existing human-caused climate change and more to come, unless we can quickly reduce our greenhouse gas emissions.
__________________________________________________________________________________
Andrew Dowdy, Principal Research Scientist in Extreme Weather, The University of Melbourne; Conrad Wasko, ARC DECRA Fellow in Hydrology, University of Sydney; Jennifer Catto, Associate Professor of Mathematics and Statistics, University of Exeter, and Seth Westra, Hydrologist, University of Adelaide
This article is republished from The Conversation under a Creative Commons license. Read the original article.
First published 19 September 2023, in Environmental Research Letters 18; reproduced here under Creative Commons Attribution 4.0 licence
Authors:
-
Giorgia Di Capua:Magdeburg-Stendal University of Applied Sciences, 39114 Magdeburg, Germany
-
Stefan Rahmstorf: Potsdam Institute for Climate Impact Research (PIK), Member of the Leibniz Association, PO Box 6012 03, D-14412 Potsdam, Germany | E-mail: [email protected]
Abstract
Extreme weather events are rising at a pace which exceeds expectations based on thermodynamic arguments only, changing the way we perceive our climate system and climate change issues. Every year, heatwaves, floods and wildfires, bring death and devastation worldwide, increasing the evidence about the role of anthropogenic climate change in the increase of extremes. In this viewpoint article, we summarize some of the most recent extremes and put them in the context of the most recent research on atmospheric and climate sciences, especially focusing on changes in thermodynamics and dynamics of the atmosphere. While some changes in extremes are to be expected and are clearly attributable to rising greenhouse gas emissions, other seem counterintuitive, highlighting the need for further research in the field. In this context, research on changes in atmospheric dynamics plays a crucial role in explaining some of these extremes and more needs to be done to improve our understanding of the physical mechanisms involved.
_______________________________________
A rise in weather extremes is probably the most severe early impact of rising global temperatures. Extreme weather events have a high impact on both human activities and natural ecosystems. In recent decades the world has seen an increase in heatwaves, droughts, flooding events and fire weather conditions, as expected in a warming climate. 2022 has been no exception, with record-breaking high temperatures registered across large portions of Western Europe, China and Pakistan (Hausfather 2023). In Pakistan, extreme monsoon rains and Himalayan glaciers melting away in a heat wave have flooded approximately 85 000 km2 (USAID 2022), killing more than 1700 people and causing $15 billion of damage (World Bank), while the Horn of Africa has seen an unprecedented drought that has pushed more than three million people into emergency food insecurity (NASA Earth Observatory 2022). Many of these events have been made more likely by anthropogenic warming and represent a serious threat to human society, with effects ranging from health and mortal- ity to economy and national security issues.
Even for lay persons it will be obvious that heat extremes will increase in a warming world. But it may be unexpected by how much: monthly heat extremes
that were three standard deviations above average during the baseline period 1951–1980 have already increased over 90-fold in frequency over the global land area, while the formerly near-unprecedented 4-sigma events have increased 1000-fold to affect 3% of the land area in any given month, data show (Robinson et al 2021). July 2023 was the hot- test month on record on Earth by a large margin (Copernicus 2023a), and likely the hottest for at least 10 000 years. Marine heatwaves have also doubled in the last few decades, and they are expected to see a 23-fold increase under a 2 ◦C warming scenario (Frölicher et al 2018)—the off-the charts sea surface temperatures in the North Atlantic in summer 2023 are a stern foretaste of this (Copernicus 2023b), while in Florida the marine heatwave caused wide spread bleaching turning into one of the worst events in the region on record (Dennis et al 2023).
Part of this is as expected simply by shifting a Gaussian normal distribution towards warmer values; the more extreme an event, the larger is the factor by which its likelihood increases. However, explaining the full extent of the global increase in extreme heat requires additional, dynamical effects (which we will discuss below).
And heat—especially lasting heat—is a silent killer. The death toll of the 2003 European heat wave has been estimated as ∼70 000 (Robine et al 2008), with a mortality peak in France higher than dur- ing any Covid19 wave (Ferrer and Breteau 2020). A first estimate for summer 2022 came in at ∼62 000 (Ballester et al 2023), when even in Britain temperatures soared above 40◦C for the first time in history. Temperature-related excess mortality is expected to increase with unmitigated global warming, even when accounting for a decrease in cold-related deaths (Gasparrini et al 2017).
Since early 2012, the fingerprint of climate change can be detected in any single day in the observed record. There are no more days on Earth where global weather is not significantly different from what it would be without human influence—on nearly all days even when just considering the weather pat- terns without the increase in global-mean temperature (Sippel et al 2020). Observations show that nearly the entire Earth surface has warmed since the late 19th century (except for the prominent ‘warming hole’ south of Greenland and Iceland, Rahmstorf et al 2015) and the annual global mean temperature has increased by 1.2◦C since the late 19th Century (Intergovernmental Panel on Climate Change 2021). The intensity and speed of warming differ by region, with land areas warming twice as much as the ocean surface since 1970 (Intergovernmental Panel on Climate Change 2021). Warming is more prominent in the Arctic, Eastern Europe, North Africa, the Middle East, East Asia and western North America. Key heatwave characteristics, such as frequency, duration and cumulative heat (i.e. the heat produced by heatwaves days inside a season), show increasing trends since 1950 at global scale, with stronger trends in tropical and northern latitudes. Trends for these key variables have been accelerating in the past few decades (Perkins-Kirkpatrick and Lewis 2020). Summer 2023 was no exception, with record breaking temperature recorded in Southeastern US, China, Spain, Morocco, rendered more likely by current climate change (Zachariah et al 2023).
While the world-wide rise in heat extremes is easily understood given the rise in global mean temperature, mean global rainfall and extreme precipitation trends depict a more complex relationship. Global rainfall is expected to increase as evaporation from warmer oceans increases. Indeed, the IPCC AR6 WGI (2021) reports an increase in globally averaged land precipitation since 1950, though with medium confidence given the large variability and spatial heterogeneity of precipitation. However, extreme rainfall events have shown a steep increase in the last few decades (especially in tropical regions), with 1 in 4 record breaking rainfall events being attributable to climate change (Robinson et al 2021). In August 2023, the region surrounding Beijing was hit by a severe flood event which saw the highest rainfall record of
the last 140 years (744.8 mm in less the 4 d) (Hawkins 2023) Perhaps counterintuitively, some areas even show opposite trends in mean precipitation rates and extreme rainfall events. One such example is the Indian summer monsoon system, which shows a slight decrease in its mean seasonal precipitation rates together with a three-fold increase in extreme rainfall events during the 1950–2015 period (Roxy et al 2017). Therefore, when considering the effect of climate change on extremes, it is not enough to look at trends in mean values.
Another example of that is that despite the global-mean (and often also local) rainfall increase, the frequency and severity of droughts has also increased in some regions, for a number of reasons. One overall reason is that with approximately constant relative humidity, air will contain (and at some point rain out) 7% more moisture per degree of warming, while the resupply of water via evaporation increases only by 2%–3% per degree (Allan et al 2020). The additional evaporation and rain- fall tends to end up in heavy rain rather than alleviating drought: Half of it comes down in the wet- test 6 d each year (Pendergrass and Knutti 2018), and the heaviest rainfall events increase most strongly (Fischer and Knutti 2015). Also, increasing agricultural and ecological droughts (i.e. loss of soil moisture and drying vegetation) can be caused not just by declining precipitation but also by rising temperatures causing faster evapotranspiration. In different regions, either of these effects can be the more important one (Cook et al 2018). Major droughts can also result from natural climate variability and are rare events (compared to the length of available observational data). Thus, studying their trend and, more importantly, attributing them to anthropogenic global warming is not an easy task (Cook et al 2018). Several regions in the world have shown an increase in drought risk, such as Western North America, the Mediterranean, East Southern Africa, East Asia and South Australia, at least partly attributable to anthropogenic warming, with the Mediterranean and the North-Western North America showing the highest confidence (Intergovernmental Panel on Climate Change 2021).
In the past decade, wildfire activity has produced some new extreme fires that are unprecedented regarding propagation speed, intensity, location, timing and burnt area (Descals et al 2022, Senande- Rivera et al 2022). For example, the Australian ‘Black Summer’ wildfire disaster in 2019/2020 fol- lowed Australia’s hottest and driest year on record, burned more than half of the habitat for over 1600 native species and directly caused 33 human deaths and almost 450 more from smoke inhalation (Himbrechts 2021). An increase in extreme fire weather conditions can already be detected at global scale, although trend magnitude and spatial patterns vary at regional scale (Jain et al 2022). Globally, anthropogenic global warming is projected to cause unprecedented increases in extreme fire weather risk in the 21st century (Touma et al 2021). Model experiments estimate that anthropogenic induced changes in fire weather indices have already emerged from natural variability for 22% of the burnable land area globally by 2019, while by the mid twenty-first century the emergence will reach 30%–60% (Abatzoglou et al 2019). Meanwhile, in August 2023, Hawaii has experienced its worst wildfire on record, and one of the worst in US history, where dry conditions and hurricane-force winds fueled the flames bringing utter devastation to the town of Lahaina, killing at least 93 people and thus surpassing the death toll of the Camp fire in California in 2018 (Gabbat and Anguiano 2023).
While warm extremes are to be expected due to anthropogenic global warming, cold extreme are projected to decrease in this century. Nevertheless, despite the general increase in global surface temperatures, a few regions show a cooling trend in the historical record. One such example is central Siberia, which features a cooling trend during boreal winter (Inoue et al 2012). While both natural variability and anthropogenic forcing are debated as causes of this trend (Inoue et al 2012), the cooling trend is mainly associated with an increase in cold extremes over the region. Cold air outbreaks in central Siberia and North America have been shown to result from sudden stratospheric warming events and a disruption of the stratospheric Polar vortex (Kretschmer et al 2018).
Detecting climate change signals can be challenging depending on the region and the variable selected, and attributing trends in temperature or precipitation fields to changes in thermodynamic or dynamic features of the atmosphere represent an even greater challenge (Shepherd 2014). In general, the largest portion of the change is to be attributed to thermodynamic effects. However, dynamic changes can further exacerbate thermodynamic driven changes and atmosphere dynamics and changes in weather patterns play an important role at regional scale (Rousi et al 2022). Amplified Rossby waves with preferred phase position, in particular waves with wave numbers 5 and 7, can lead to concurrent heatwaves (and crop failures) in the mid-latitudes (Kornhuber et al 2020), raising concerns about future food security. Arctic amplification, despite being more prominent in winter than summer, may also affect westerly winds, storm tracks and wave-guides in the mid-latitudes (Coumou et al 2018). Analyzing the ability of models to reproduce amplified waves 5 and 7 shows that even a small bias in upper tropospheric circulation features can have a strong impact on surface temperature and rainfall patterns (Luo et al 2022), highlighting that it is difficult for global climate models to simulate all mechanisms that contribute to making weather more extreme.
Europe has emerged as a hot-spot of heat extremes: it has seen a stronger increase in summer heat than other regions in the northern mid-latitudes. This enhanced warming has been related to dynamical changes such as an increase of double jet patterns, which could explain all of the additional rise in heat waves beyond what is expected simply by thermodynamics (Rousi et al 2022). Both observation and model experiment support the hypothesis that shrinking Arctic sea ice and reduced snow cover over northern Eurasia in spring can also contribute to increased blocking over Europe and consequent frequency of heatwaves (Zhang et al 2020). Sea surface temperature anomalies (in particular the northern Atlantic ‘warming hole’ mentioned earlier) can also reinforce heatwaves in central Europe, such as in 2015 (Duchez et al 2015).
In summary, it is now clear that global warming is already greatly increasing the number and intensity of many types of weather extremes, as has been predicted by climate science for decades. Much of this is due to thermodynamics. With that we mean that the atmosphere is warmer, which means it holds more energy and water to power extreme weather. The ocean is also warmer and can provide more energy and moisture as fuel to tropical cyclones. However, increasingly the attention of researchers has turned to dynamic effects. With that we refer to changes in circulation and stability of atmosphere and ocean. It includes changes to the jet stream, polar vortex, atmospheric planetary waves or to the Atlantic meridional overturning circulation.
Obtaining robust conclusions about changes in weather extremes requires long time series, given that extreme events are by definition rare events and are not easy to model. Nevertheless, the signal of climate change has now clearly emerged from the noise for many types of extremes. Disentangling the dynamic mechanisms is harder again and represents a current frontline of research. Driving forces behind dynamic changes are often regionally diverse temperature changes, such as Arctic amplification, enhanced land warming and sea surface temperature anomalies. Many facets of the dynamic mechanisms are still being debated in the scientific literature. Even if not everything is fully understood, researchers and journalists should not be shy to use every opportunity to educate the public about the fact that human-caused global warming is making weather extremes worse, already causing serious harm to many millions of people.
Even once global warming is stopped, we will see unprecedented extremes for a long time to come. Just think of a former once-in-5000 year event which at 1.5◦C warming may have become a once-in-50 year event. Thus, it will take many decades until we have seen all the possible extreme events a 1.5◦C warmer world has in store for us.
References
Abatzoglou J T, Williams A P and Barbero R 2019 Global emergence of anthropogenic climate change in fire weather indices Geophys. Res. Lett. 46 326–36
Allan R P et al 2020 Advances in understanding large-scale responses of the water cycle to climate change Ann. New York Acad. Sci. 1472 49–75
Ballester J, Quijal-zamorano M, Fernando R, Turrubiates M, Pegenaute F, Herrmann F R, Robine J M and Basagan ̃a X 2023 Heat-related mortality in Europe during the summer of 2022 Nat. Med. 29 1857–66
Cook B I, Mankin J S and Anchukaitis K J 2018 Climate change and drought: from past to future Curr. Clim. Change Rep. 4 164–79
Copernicus 2023a July 2023 sees multiple global temperature records broken Copernicus (available at: https://climate. copernicus.eu/july-2023-sees-multiple-global-temperature- records-broken)
Copernicus 2023b Record-breaking North Atlantic Ocean temperatures contribute to extreme marine heatwaves Copernicus Online (available at: https://climate.copernicus. eu/record-breaking-north-atlantic-ocean-temperatures- contribute-extreme-marine-heatwaves)
Coumou D, Di Capua G, Vavrus S, Wang L and Wang S 2018 The influence of Arctic amplification on mid-latitude summer circulation Nat. Commun. 9 2959
Dennis B, Ajasa A and Mooney C 2023 As Florida ocean temperatures soar, a race to salvage imperiled corals (Washington Post) (available at: www.washingtonpost.com/ climate-environment/2023/07/26/florida-coral-reef-ocean- temperatures-heat/)
Descals A, Gaveau D L A, Verger A, Sheil D, Naito D and Pen ̃uelas J 2022 Unprecedented fire activity above the Arctic circle linked to rising temperatures Science 378 532–7
Duchez A, Frajka-williams E, Josey S A, Evans D G, Grist J P, Marsh R, McCarthy G D, Sinha B, Berry D I and Hirschi J J-M 2015 Drivers of exceptionally cold North Atlantic Ocean temperatures and their link to the 2015 European heat wave Environ. Res. Lett. 11 1–9
Ferrer M and Breteau P 2020 Coronavirus: un pic très net de mortalité en France enregistré en mars et avril Le Monde (available at: www.lemonde.fr/les-decodeurs/article/2020/ 04/27/coronavirus-un-pic-tres-net-de-mortalite-en-france- depuis-le-1er-mars-par-rapport-aux-vingt-dernieres- annees_6037912_4355770.html)
Fischer E M and Knutti R 2015 Anthropogenic contribution to global occurrence of heavy-precipitation and high-temperature extremes Nat. Clim. Change 5 560–4
Frölicher T L, Fischer E M and Gruber N 2018 Marine heatwaves under global warming Nature 560 360–4
Gabbat A and Anguiano D 2023 Hawaii wildfires: deadliest US blaze in a century kills at least 93 people Guard (available at: www.theguardian.com/us-news/2023/aug/13/hawaii- wildfires-at-least-89-confirmed-killed-after-deadliest-us- blaze-in-100-years)
Gasparrini A et al 2017 Projections of temperature-related excess mortality under climate change scenarios Lancet Planet. Health 1 e360–7
Hausfather Z 2023 State of the climate: how the world warmed in 2022 Carbon Brief (available at: www.carbonbrief.org/state- of-the-climate-how-the-world-warmed-in-2022/)
Hawkins A 2023 Chinese firefighter ‘dies heroic death’ as Beijing reports heaviest rain in 140 years Guard (available at: www. theguardian.com/world/2023/aug/02/beijing-reports- heaviest-rain-140-years-china-g20-climate-talks)
Himbrechts D 2021 Australia’s Black Summer of fire was not normal—and we can prove it The Conversation (available at: https://theconversation.com/australias-black-summer-of- fire-was-not-normal-and-we-can-prove-it-172506)
Inoue J, Hori M E and Takaya K 2012 The role of Barents Sea ice in the wintertime cyclone track and emergenceof a warm-Arctic cold-Siberian anomaly J. Clim. 25 2561–9
IPCC 2021 Climate Change 2021: The Physical Science Basis.
Contribution of Working Group I to the Sixth Assessment Report of the Intergovernmental Panel on Climate Change ed V Masson-Delmotte et al (Cambridge University Press) (https://doi.org/10.1017/9781009157896)
Jain P, Castellanos-Acuna D and Coogan S C P 2022 Observed increases in extreme fire weather driven by atmospheric humidity and temperature Nat. Clim. Chang. 12 63–70
Kornhuber K, Coumou D, Vogel E, Lesk C, Donges J F, Lehmann J and Horton R M 2020 Amplified Rossby waves enhance risk of concurrent heatwaves in major breadbasket regions Nat. Clim. Change 20 48–53
Kretschmer M, Cohen J, Matthias V, Runge J and Coumou D 2018 The different stratospheric influence on cold-extremes in Eurasia and North America npj Clim. Atmos. Sci. 1 1–10
Luo F et al 2022 Summertime Rossby waves in climate models: substantial biases in surface imprint associated with small biases in upper-level circulation Weather Clim. Dyn.
3 905–35
NASA Earth Observatory 2022 Worst Drought on Record Parches Horn of Africa (available at: https://earthobservatory.nasa. gov/images/150712/worst-drought-on-reco)
Pendergrass A G and Knutti R 2018 The uneven nature of daily precipitation and its change Geophys. Res. Lett.
45 980–11,988
Perkins-Kirkpatrick S E and Lewis S C 2020 Increasing trends in regional heatwaves Nat. Commun. 11 1–8
Rahmstorf S, Box J E, Feulner G, Mann M E, Robinson A, Rutherford S, Scha E J and Schaffernicht E J 2015 Exceptional twentieth-century slowdown in Atlantic Ocean overturning circulation Nat. Clim. Change 5 475–80
Robine J M, Cheung S L K, Le Roy S, Van Oyen H, Griffiths C, Michel J P and Herrmann F R 2008 Death toll exceeded 70,000 in Europe during the summer of 2003 C. R. Biol. 331 171–8
Robinson A, Lehmann J, Barriopedro D, Rahmstorf S and Coumou D 2021 Increasing heat and rainfall extremes now far outside the historical climate npj Clim. Atmos. Sci. 4 3–6
Rousi E, Kornhuber K, Beobide-Arsuaga G, Luo F and Coumou D 2022 Accelerated western European heatwave trends linked to more-persistent double jets over Eurasia Nat. Commun. 13 1–11
Roxy M K, Ghosh S, Pathak A, Athulya R, Mujumdar M, Murtugudde R, Terray P and Rajeevan M 2017 A threefold rise in widespread extreme rain events over central India Nat. Commun. 8 1–11
Senande-Rivera M, Insua-Costa D and Miguez-Macho G 2022 Spatial and temporal expansion of global wildland fire activity in response to climate change Nat. Commun.
13 1–9
Shepherd T G 2014 Atmospheric circulation as a source of uncertainty in climate change projections Nat. Geosci. 7 703–8
Sippel S, Meinshausen N, Fischer E M, Székely E and Knutti R 2020 Climate change now detectable from any single day of weather at global scale Nat. Clim. Change 10 35–41
Touma D, Stevenson S, Lehner F and Coats S 2021 Human-driven greenhouse gas and aerosol emissions cause distinct regional impacts on extreme fire weather Nat. Commun. 12 1–8
USAID 2022 Pakistan Flood Fact Sheet (available at: www.usaid. gov/sites/default/files/2022-12/2022-09- 30_USG_Pakistan_Floods_Fact_Sheet_8.pdf)
Zachariah M, Philip S, Pinto I, Vahlberg M, Singh R, Arrighi J R, Barne C and Otto F E L 2023 Extreme heat in North America, Europe and China in July 2023 made much more likely by climate change (https://doi.org/10.25561/105549)
Zhang R, Sun C, Zhu J, Zhang R and Li W 2020 Increased European heat waves in recent decades in response to shrinking Arctic sea ice and Eurasian snow cover npj Clim. Atmos. Sci. 3 7
New extreme records have already been set for ocean temperatures. Not looking forward to what this means for ecosystems and extreme weather events around Aotearoa:
From Copernicus European Space Agency Climate Change Service; read the full report here.
Global surface air temperature highlights:
- 2023 is confirmed as the warmest calendar year in global temperature data records going back to 1850
- 2023 had a global average temperature of 14.98°C, 0.17°C higher than the previous highest annual value in 2016
- 2023 was 0.60°C warmer than the 1991-2020 average and 1.48°C warmer than the 1850-1900 pre-industrial level
- It is likely that a 12-month period ending in January or February 2024 will exceed 1.5°C above the pre-industrial level
- 2023 marks the first time on record that every day within a year has exceeded 1°C above the 1850-1900 pre-industrial level. Close to 50% of days were more than 1.5°C warmer then the 1850-1900 level, and two days in November were, for the first time, more than 2°C warmer.
- Annual average air temperatures were the warmest on record, or close to the warmest, over sizeable parts of all ocean basins and all continents except Australia
- Each month from June to December in 2023 was warmer than the corresponding month in any previous year
- July and August 2023 were the warmest two months on record. Boreal summer (June-August) was also the warmest season on record
- September 2023 was the month with a temperature deviation above the 1991–2020 average larger than any month in the ERA5 dataset
- December 2023 was the warmest December on record globally, with an average temperature of 13.51°C, 0.85°C above the 1991-2020 average and 1.78°C above the 1850-1900 level for the month. You can access information specific for December 2023 in our monthly bulletin